Photovoltaics: Highly relevant basic research
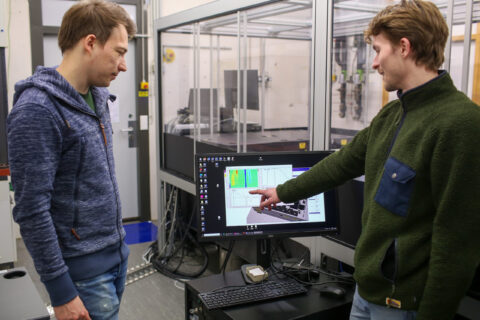
Phillip Greißel and Dominik Thiel are researching particularly efficient solar cells
At the current time we are still burning vast amounts of fossil fuels such as crude oil or coal in a bid to quench our thirst for energy. This generates the greenhouse gas carbon dioxide that acts like a warming woolen blanket in the atmosphere. It is the main reason for the temperature on Earth rising year by year. If we want to slow down climate change, we have to change the way we produce energy. An important role is played by photovoltaic units that use sunlight to generate electricity. The chemists Phillip Greißel and Dominik Thiel from Friedrich-Alexander-Universität Erlangen-Nürnberg (FAU) are investigating how to optimize this process. Their findings may lead to the development of a new generation of solar cells that are considerably more efficient than current models. We spoke to the scientists.
Mr. Greißel, Mr. Thiel, today’s solar cells convert at most one quarter of solar energy into electrical energy. Why are they so inefficient?
Dominik Thiel: There are several reasons. One is that a major part of the energy that shines on to the solar cell is lost as heat. In order to understand that, it is first of all important to know that solar cells are made of semiconducting materials such as silicon. These usually conduct electricity very poorly. Their very nature means that there are no freely movable charge carriers inside them, but these are required for electricity to flow. The electrons that may usually be available are all required for bonds between the atoms in the solar cell.
And that changes when light hits the cell?
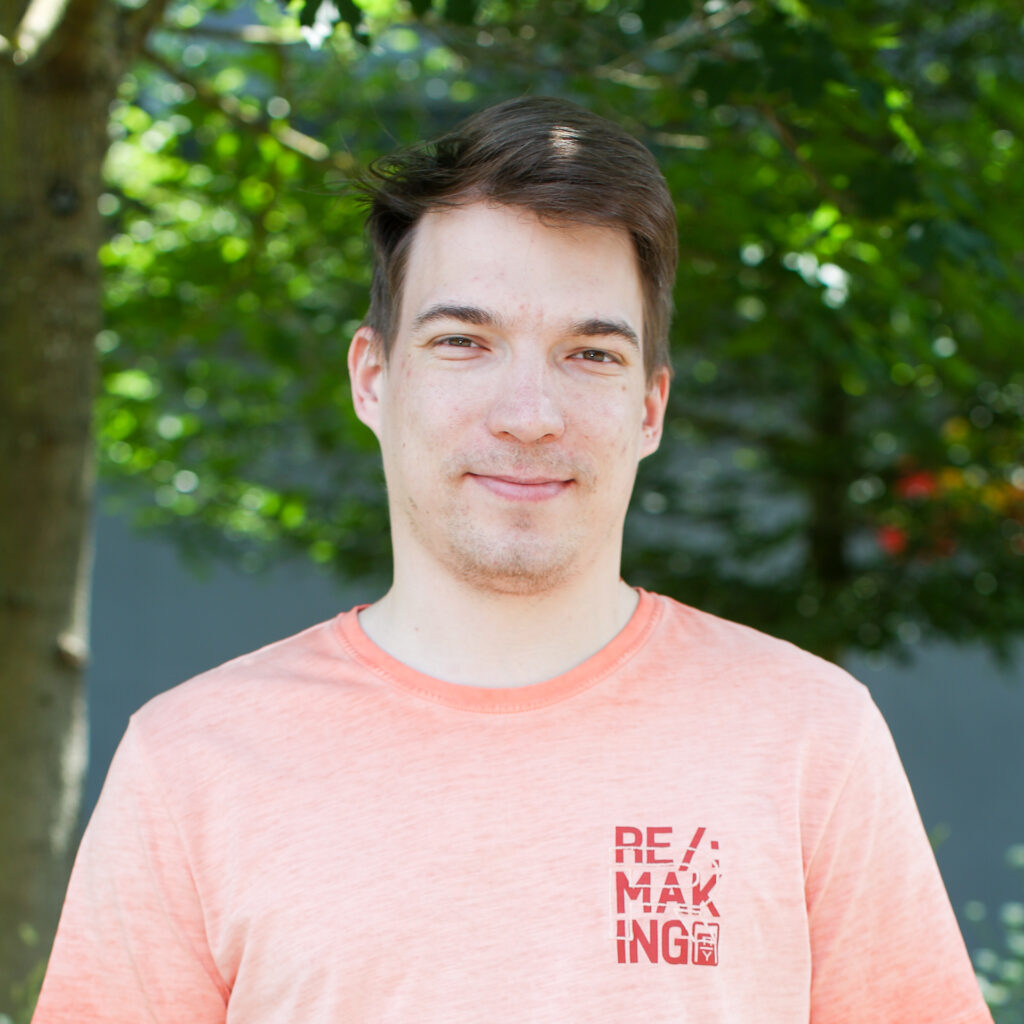
Dominik Thiel: That’s right. If a light particle, a photon, hits a semiconductor atom it can excite one of the electrons. The electron then more or less breaks loose and jumps from the valence band into what is known as the conduction band. This creates an electrical charge that can be used, for example, to drive a motor or boil water. However, electrons require a certain minimum excitation energy before they can jump into the conduction band. Otherwise they are not able to move across the band gap.
If a photon does not have enough energy, the nudge it gives the electrons is too weak and the conduction band remains empty?
Phillip Greißel: That’s right. The amount of energy light has depends on its color: red light for example has less energy than yellow and yellow light has less energy than blue. Presuming you have a solar cell whose band gap is so wide that yellow light is required to cross over it. Red light will be of no benefit to this solar cell, as its energy is too low. Light of this color does not produce an electrical charge, no matter how bright the light is. A brighter light just means that there are more “red” photons, but not that their energy is higher. And a red photon does not have enough energy to excite an electron. In this example, the red photons are basically “lost”.
Dominik Thiel: A “blue” photon would have more than enough energy to cross over the band gap, however. And this surplus energy is usually emitted as heat. That is the case even if the light is so high in energy that it would in theory be sufficient to move two electrons over the band gap. It always just excites one electron and the surplus energy is released as heat. These losses mean that traditional solar cells cannot reach rates of efficiency of more than 33 percent even under optimal circumstances. That is the maximum possible rate of efficiency. Standard cells available on the market today usually offer 22 percent, so considerably less.
However, there are approaches which could in theory lead to rates of 45 percent. What do they involve?
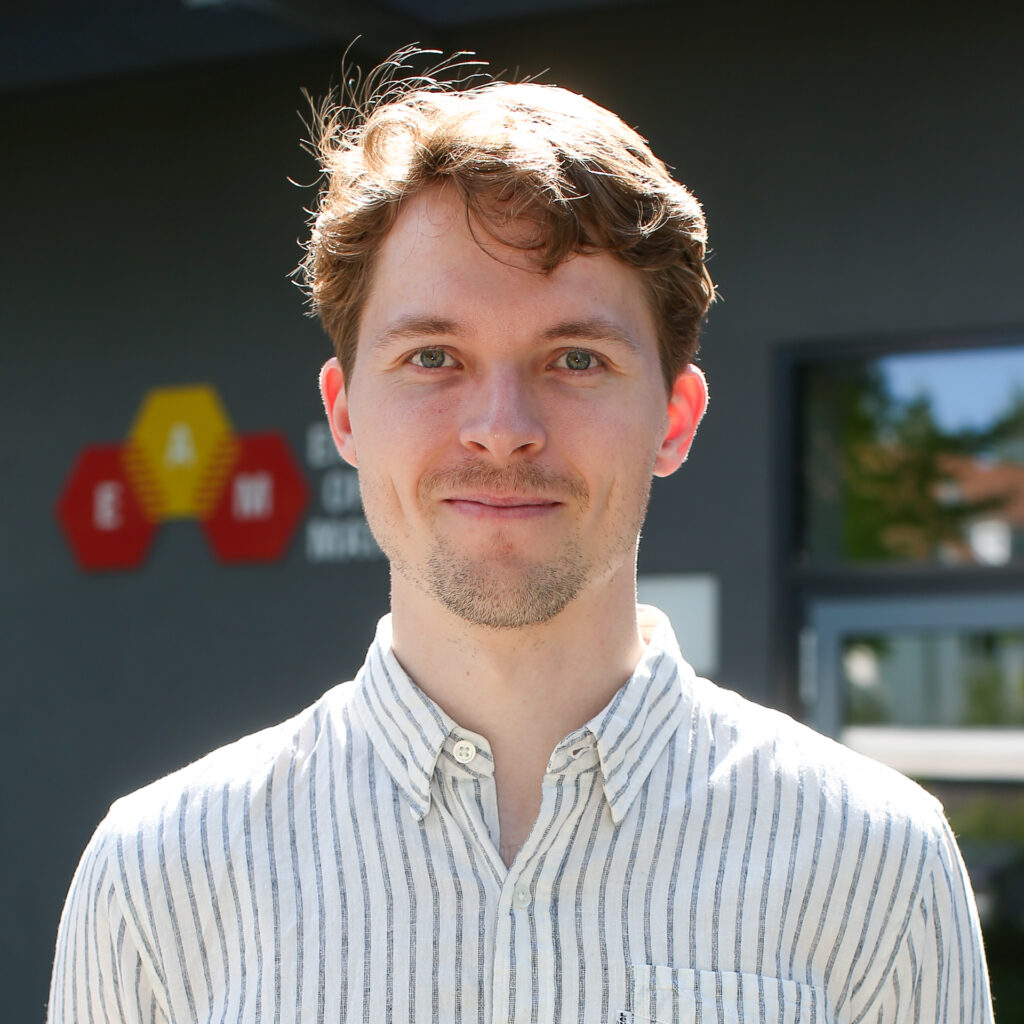
Phillip Greißel: That is the very topic that we are exploring in Prof. Dr. Dirk Guldi’s working group at the Chair of Physical Chemistry under the auspices of the FAU Profile Center Solar. We are trying to make use of the surplus energy so that a high-energy photon can generate two free charge carriers, not just one. This is an area that interests numerous scientists across the globe. It is based on a method known as “singlet fission”, where a high-energy singlet excited electronic state is converted into two lower-energy excited electronic states. These two new excited states must then be stabilized before they can be used to generate free charge carriers. We have already achieved some promising results by using specific molecules.
Which molecules have you used?
Dominik Thiel: In our latest study we used a compound of six identical molecules, a hexamer. This allowed the two lower energy excited states to be achieved very rapidly. At the same time, the hexamer structure meant that they were able to each move to different positions in the compound due to diffusion. This separation made them considerably more stable. The two goals of “rapid formation” and “high stability” are contradictory. However, they are both important if we are to make efficient use of high-energy light to produce free charge carriers.
How do you know which compounds are suitable? I presume you don’t follow the “trial and error” approach?
Dominik Thiel: No, we don’t. We work with fellow researchers from theoretical chemistry. They can calculate, for example, which characteristics molecules should have in order to conduct singlet fission after being excited by light of a certain energy. We then take these results and ask groups from organic chemistry to synthesize molecules accordingly. If the synthesis is successful, we test the compounds and try to gain a better understanding of their behavior which we can then use to optimize them further, working once more in collaboration with the theorists.
Phillip Greißel: That is an aspect that fascinates us both: collaboration between different branches of chemistry that finally leads to us obtaining a molecule that has exactly the properties we expect from it. It is great feeling when everything works out, especially when it concerns such a relevant task as finding new ways of gaining energy in future.
When will the first solar cell based on your compounds become available?
Phillip Greißel: Certainly not any time in the near future, it is still at the stage of basic research. In order to obtain a real solar cell we would have to combine our compounds with a suitable semiconductor. At present, our molecules are only compatible with a select few exotic semiconductor materials. However, it is likely that the principle behind them can be transferred to other compounds that may then be suitable for use in traditional silicon solar cells. In addition, some of our colleagues have recently demonstrated that our singlet fission molecules really can convert light to electrical energy in the laboratory. There is still a long way to go before it is ready for the mass market, though.
What is next in the pipeline for you both?
Dominik Thiel: I am coming to the end of my doctoral degree and have been wondering for a while whether to continue to pursue a career in academia or not. I have come to the conclusion that I would rather work in the research and development department of a company because it is more application-oriented. I could also imagine working for a research institute with close ties to industry, that would bridge the gap between both worlds.
Phillip Greißel: I would like to stay in academia, particularly the area of regenerative energy, but I am also open to other areas. Luckily, chemistry is a very diverse subject, and that is one of the reasons I like it so much. The question of how molecules react to light is what I am particularly interested in at the moment, also due to the highly interesting laser optic methods that we are using to test our compounds.
Further information:
Dominik Thiel
Chair of Physical Chemistry I
dominik.dt.thiel@fau.de
Phillip Greißel
Chair of Physical Chemistry I
phillip.greissel@fau.de